Optimize Production with Precision Aquaculture
Increasing feed and energy costs. Environmental threats. Production inefficiencies. These are just a few of the challenges fish farms face each day.
Innovasea’s precision aquaculture technologies are here to help. They enable aquaculture operators to closely monitor, manage and control their operations – pen-by-pen and across sites – based on reliable real-time data. It all adds up to better decision-making so your farm can boost profits and become more sustainable.
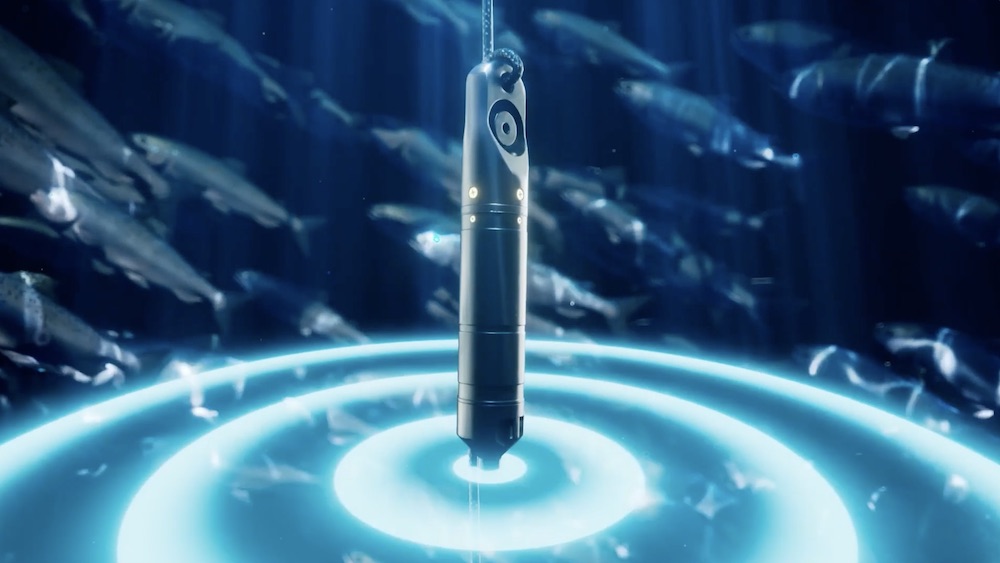
The Future of Fish Farming
Fish farming is entering a new era, one where day-to-day decisions need to be based on empirical data and analytics rather than past practice, gut instinct and best guesses. By embracing new tools and technologies to better understand their complex operations, today’s farmers are saving money, reducing mortalities and becoming more efficient.
Using a mix of sophisticated sensors, high resolution cameras, artificial intelligence and cloud computing, Innovasea’s integrated solutions deliver unprecedented insights into how your farm is operating – from measuring oxygen levels to ensure fish remain healthy to accurately estimating biomass to reduce feeding costs.

Scalable and Versatile
Our solutions are easily scalable so you can monitor individual fish pens or entire farms. It all adds up to better control over your farm and a deeper understanding of how to manage it – and that means healthier fish, better production and more efficient operations.
Customer Success
Already a customer? If you have a question about your equipment, please contact Customer Success:
Norway: support@innovasea.com | Canada: support-intelligence@innovasea.com |
Chile: soporte.chile@innovasea.com | Greece: support.hellas@innovasea.com
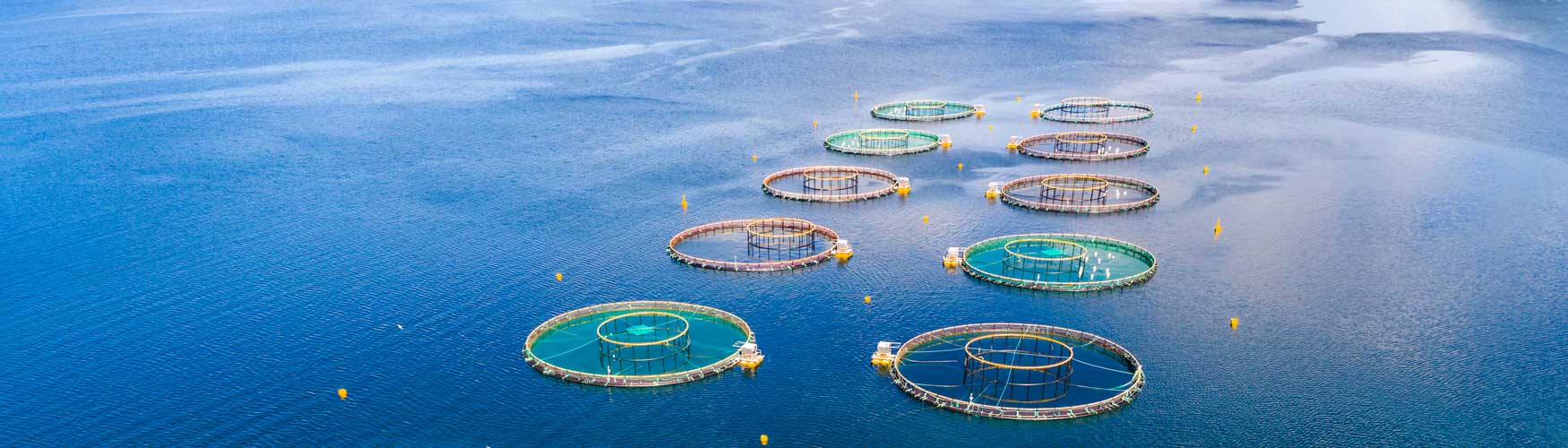
Want to learn more?
Contact Innovasea today to find out how our aquaculture experts can help with your next project.